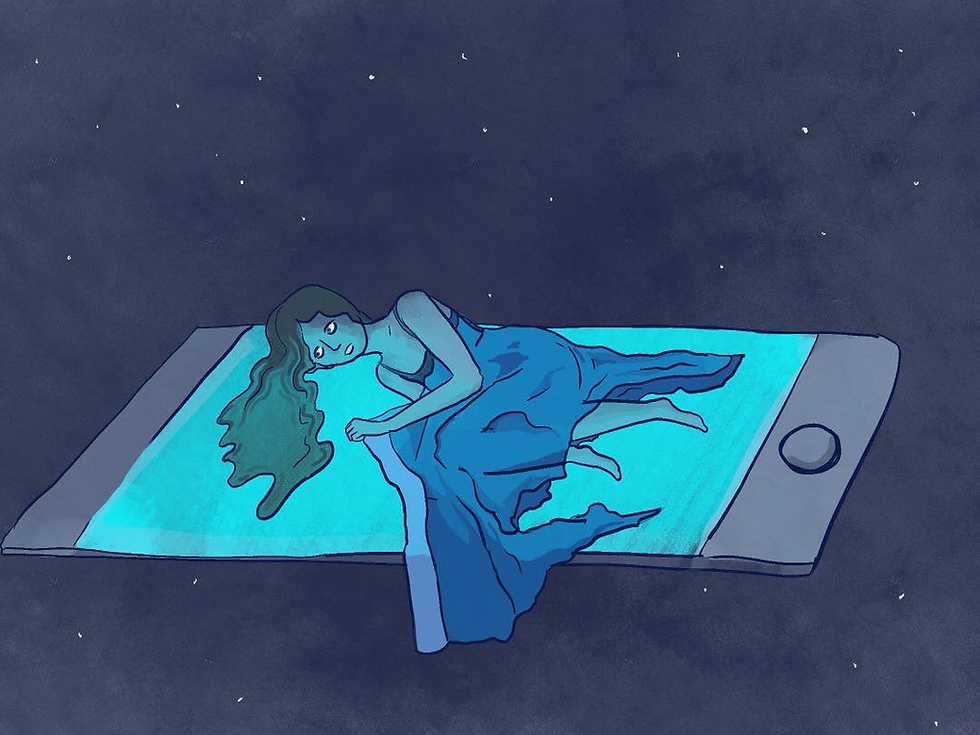
You roll over onto the other side of the bed, flip your pillow, and try to clear your mind so you can finally fall asleep. You’ve been in this sleepless scenario before with the relief of sweet dreams appearing a million sheep away. “Why can’t I sleep?” you ask yourself. “I went through my nightly routine and even watched a few extra TikTok videos to relax.” Though it may feel like a bedtime social media binge or Netflix marathon is just what you need to wind down, in reality, this routine is actually what disrupts your sleep. This is because the blue light emitted from electronic screens desynchronizes your circadian rhythm, the process responsible for regulating the sleep-wake cycle. Once we have identified blue light exposure due to late-night electronic use as a culprit behind your inability to sleep, we can identify ways in which you can minimize its effects.
Blue light’s wake-inducing rays are crucial during the daytime as many of us naturally wake up when the sun rises and feel tired when it is dark. Light exposure is one of the most important indicators that tell your brain when it’s time to be alert or that it’s time to unwind [1]. The light emitted from the sun is termed “white light” because it contains all colors of the visual light spectrum and therefore appears colorless. Each color of light contained in white light corresponds to a unique wavelength of electromagnetic radiation. Blue light corresponds to wavelengths around 480 nanometers, and is part of the light spectrum emitted from the screens of electronic devices [1]. For now, it suffices to say that light exposure in general is associated with wakefulness. As we shall see, blue light specifically has an impact on our circadian rhythms.
Blue light exposure at night is detrimental to the sleep-wake cycle because our brains use light as a cue to entrain our circadian rhythms. Circadian rhythms are approximately 24-hour cycles that are internally driven within our brains. Although they run in 24-hour cycles independently, circadian rhythms usually coincide with day and night [2]. One of the main ways that humans synchronize their circadian rhythms with the solar light-dark cycle is by detecting changes in light color, intensity, and duration with their eyes [3]. When light regulates one’s circadian rhythm, it is called photoentrainment [2]. Most human circadian rhythms that cause the sleep-wake cycle entrain themselves to the external light-dark cycle so that we sleep when it is dark and are awake when it is light outside. However, the sun isn’t the only source of light to which you are exposed. Blue light from electronic devices can interfere with the natural photoentrainment of your circadian rhythm, thus altering your sleep-wake pattern [4].
In order to understand why blue light from your phone screen during that phone binge is keeping you awake, let’s consider light as it first enters the eye. An extension of nerve cells at the back of the eye, known as the retina, contains two photoreceptors known as rods and cones. Both rods and cones use photopigments to transform the light received by your eye into signals that the brain can process [3]. Photopigments are molecules that interact with light to induce a process which causes rods and cones to send signals to cells called retinal ganglion cells (RGCs). RGCs are the messengers that transmit light information from the retina to the brain [3].
RGCs bundle together to form the optic nerve, which connects your eyes to regions of the brain [3]. The optic nerve transports signals from rods and cones to the areas in the brain responsible for vision, or image-formation. These signals are what allow you to see visual images of your surroundings, such as a sunny day or starry night. Although light reception in the eye is key for image-formation, it also has many other effects [5]. One of the most important roles of light signals emerges when RGCs send non-image-forming (NIF) signals to the brain based on light reception. This includes signals sent to the part of your brain responsible for the circadian rhythm.

The category of RGCs that are able to detect light on their own are known as intrinsically photosensitive RGCs (ipRGCs). ipRGCs detect light similarly to rods and cones, using photopigments that react to light to create their own signals [3]. ipRGCs form part of the optic nerve and thus carry information from the rods and cones to which they are connected [5]. As part of the optic nerve, ipRGCs help create visual images like other RGCs. In addition, ipRGCs also branch out from the optic nerve to non-image-forming parts of the brain, primarily those involved in the circadian rhythm. Thus, ipRGCs are a major part of entraining your sleep cycle. ipRGCs use melanopsin to detect light, which is a photopigment that is highly sensitive to blue light [1,6]. Exposure to blue light causes ipRGCs to send signals to your brain to photoentrain your circadian rhythm. Since the light emitted from electronic devices contains blue light, screen use at night can desynchronize your circadian rhythm.
The most important brain structure involved in circadian rhythms is the suprachiasmatic nucleus (SCN), which receives NIF information from ipRGCs. The SCN is located in the hypothalamus, the part of the brain responsible for maintaining homeostasis by linking the nervous and endocrine systems. It is the “master-pacemaker” of circadian rhythms, as evidenced by experiments on animals without a functioning SCN that show that they are unable to exhibit any circadian rhythms, including the sleep-wake cycle [2,4]. The SCN uses light information from ipRGCs to send signals to the pineal gland, which releases the hormone melatonin [7]. Melatonin levels increase as night creeps in, inducing the feeling of sleepiness and telling you that it’s time to rest up. However, melatonin production decreases with exposure to light, especially blue light. This is because ipRGCs use melanopsin to induce signals that travel to the SCN when exposed to blue light. These ipRGC signals tell the SCN to impede the secretion of melatonin. With limited levels of blue light, such as during the night, ipRGCs stop sending signals that suppress the release of melatonin from the pineal gland. Using electronics after dark can therefore suppress melatonin secretion, disrupting your ability to fall asleep.
Now that we understand the way in which circadian rhythms use NIF information to entrain themselves to the solar light-dark cycle, we can comprehend why blue light exposure is so disruptive to our circadian rhythms. Before we harnessed electricity, people relied on fire to illuminate their surroundings when it was dark outside, which emits longer wavelengths of light and thus little blue light. Most people naturally arose with the sun and fell asleep once it had gone down. Our current age is therefore unprecedented because 99% of Americans and Europeans are exposed to significant night-time light pollution [8]. Nearly 80% of North Americans cannot even see the Milky Way Galaxy [8]! The baseline exposure to high levels of artificial light at night compounded with bright electronics within our homes desynchronizes our circadian rhythms [8,9].
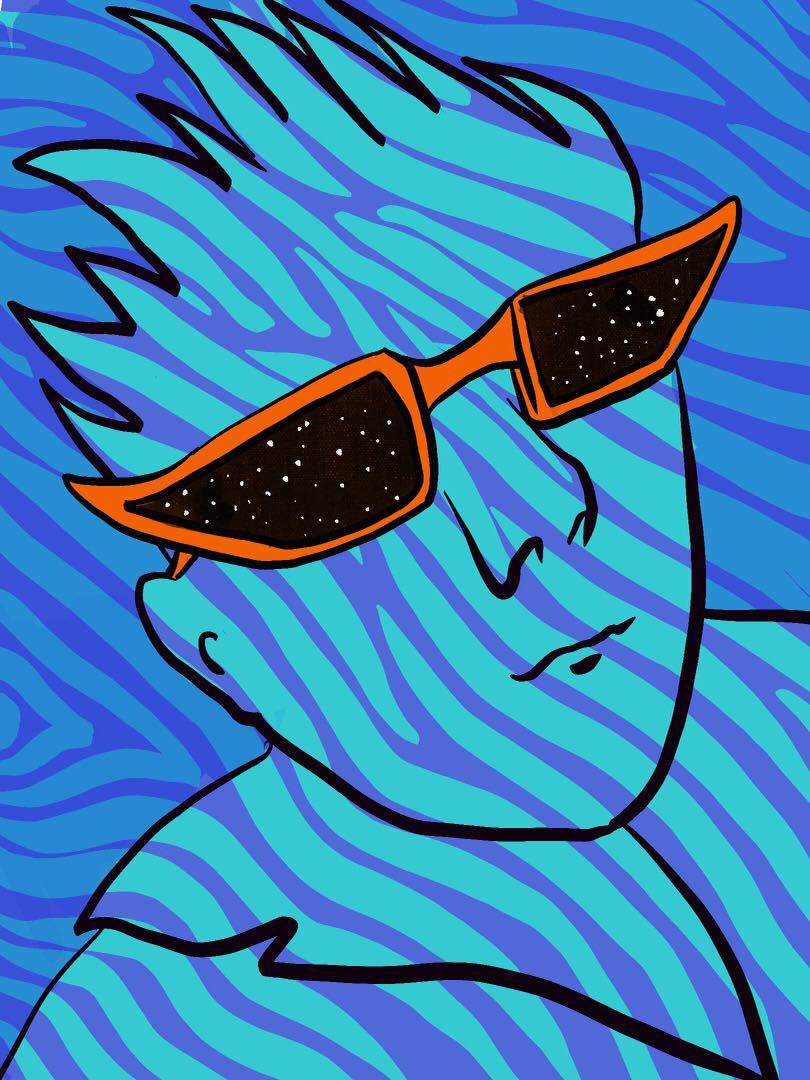
Short term exposure to light for even just about a half an hour is enough to suppress melatonin levels. Long-term exposure of 90 to 120 minutes or more of blue light at moderate levels has the greatest impact on melatonin levels [10]. One study of thirteen participants displayed that iPad use at full brightness for two hours can suppress normal melatonin levels by 23%, with additional exposure to blue light only compounding its effects [11]. The authors posit that larger screens from TVs, holding devices closer to our eyes, and additional environmental light exposure at night all suppress melatonin to an even greater extent [11].
With this information in mind, you don’t have to regress to archaic times without electronics to brighten your day (or night). There are many solutions to melatonin suppression from blue light exposure at night. Many of these solutions are practical and easy to implement. Since the melanopsin of your ipRGCs is most sensitive to blue light, you can turn your devices to “night mode,” which emits longer wavelengths, such as red and orange, and is less detrimental to your sleep [2,10]. Additionally, using devices at their minimum brightness at night decreases blue light exposure and often doesn’t greatly impact our ability to read their screens [11].
In addition to altering your electronic screen’s brightness and hue, there is a stylish solution to the disruptive effects of blue light exposure at night. The trendy solution of blue-light-blocking (BB) glasses has been proven to help minimize melatonin suppression [12]. The patients of one study who wore BB glasses from 6pm to the time they went to sleep at 11:30pm displayed significantly higher levels of melatonin, were much less alert, and displayed slower reaction times than the control group. Both the BB and control groups continued their evenings with normal activities and light exposure. Although statistically limited, the authors suggest that BB glasses do in fact combat blue light’s effects on melatonin levels, and in turn, allow wearers to feel tired when they are about to call it a night [12]. By limiting their blue light exposure before bed, the patients wearing BB glasses were able to unwind while still using electronics.
The COVID-19 pandemic forced many to turn their lifestyles completely virtual, using electronics throughout the day and night for school and work. Some of us may have adopted additional electronic use into our nighttime routines, with means such as TikTok and Netflix providing sources of comfort and relaxation. Although it is not realistic to completely eliminate electronic use after dark, there are many ways in which we can limit our exposure to blue light. By learning how screen use affects our sleep cycles, we are able to minimize the ability of blue light to desynchronize our circadian rhythms. We can now count a few less sheep to finally fall asleep.
Rüger, M., St Hilaire, M. A., Brainard, G. C., Khalsa, S. B., Kronauer, R. E., Czeisler, C. A., & Lockley, S. W. (2013). Human phase response curve to a single 6.5 h pulse of short-wavelength light. The Journal of Physiology, 591(1), 353–363. https://doi.org/10.1113/jphysiol.2012.239046
Van Gelder, R. N., & Buhr, E. D. (2016). Ocular Photoreception for Circadian Rhythm Entrainment in Mammals. Annual Review of Vision Science, 2, 153–169. https://doi.org/10.1146/annurev-vision-111815-114558
Ospri, L., Prusky, G., & Hattar, S. (2017). Mood, the Circadian System, and Melanopsin Retinal Ganglion Cells. Annual Review of Neuroscience, 40, 539–556. https://doi.org/10.1146/annurev-neuro-072116-031324
Fonken, L. K., & Nelson, R. J. (2014). The effects of light at night on circadian clocks and metabolism. Endocrine Reviews, 35(4), 648–670. https://doi.org/10.1210/er.2013-1051
Altimus, C. M., Güler, A. D., Villa, K. L., McNeill, D. S., Legates, T. A., & Hattar, S. (2008). Rods-cones and melanopsin detect light and dark to modulate sleep independent of image formation. Proceedings of the National Academy of Sciences of the United States of America, 105(50), 19998–20003.
Berson, D. M., Dunn, F. A., & Takao, M. (2002). Phototransduction by retinal ganglion cells that set the circadian clock. Science, 295(5557), 1070–1073. https://doi.org/10.1126/science.1067262
NCCIH. (2021, January). Melatonin: What You Need to Know. U.S. Department of Health and Human Services. https://www.nccih.nih.gov/health/melatonin-what-you-need-to-know#:~:text=Melatonin%20is%20a%20hormone%20that,in%20the%20body%20beyond%20sleep.
Walker, W.H., Walton, J.C., DeVrries, A.C., & Nelson, R.J. (2020). Circadian rhythm disruption and mental health. Translational Psychiatry, 10(28), n.p. https://doi.org/10.1038/s41398-020-0694-0
Oh, Ji Hye et al. “Analysis of circadian properties and healthy levels of blue light from
smartphones at night.” Scientific reports vol. 5 11325. 18 Jun. 2015, doi:10.1038/srep11325
Tähkämö, L., Partonen, T., & Pesonen, A. K. (2019). Systematic review of light exposure impact on human circadian rhythm. Chronobiology International, 36(2), 151-170, https://doi.org/10.1080/07420528.2018.1527773
Wood, B., Rea, M.S, Plitnick, B., & Figueiro, M.G. (2012). Light level and duration of exposure determine the impact of self-luminous tablets on melatonin suppression. Applied Ergonomics, 44, 237-240. http://dx.doi.org/10.1016/j.apergo.2012.07.008
Cajochen, C., Bromundt, V., & Schmidt, C. (2015). Blue blocker glasses as a countermeasure for alerting effects of evening light-emitting diode screen exposure in male teenagers. The Journal of Adolescent Health : Official Publication of the Society for Adolescent Medicine, 56(1), 113–119. https://doi.org/10.1016/j.jadohealth.2014.08.002
Comments